Big Ideas
CONTEXT
The myriad of global stressors facing marine ecosystems can be acutely exacerbated by the harmful effects of invasive and irruptive species. These synergistic stresses compound the need for novel strategies to control these species. Invasive species generally refer to non-native species that are impacting a marine ecosystem. A species irruption is a sudden change in the population density of a species, typically characterized by population explosions followed by subsequent crashes (Roughgarden 1996). Invasions and irruptions can have many negative effects on ecosystems:
- displace native species (e.g. Huxel 1999),
- cause the loss of native genotypes (e.g. Gurevitch & Padilla 2004),
- lead to a reduction in biodiversity,
- modify habitats, change community structure, affect food-web properties and ecosystem processes (Byrnes et al. 2007; D’eath et al. 2014),
- impede the provision of ecosystem services (e.g. Katsanevakis et al., 2014),
- impact human health (Pyšek & Richardson 2010), and
- cause substantial economic harm (Marbuagh et al. 2014).
Rapid globalization and increasing trade, travel, and transport are accelerating rates of new introductions through various pathways, such as shipping (ballast water), navigational canals, aquaculture, and the aquarium trade (Molnar et al. 2008; Riccardi et al. 2017). Furthermore, climate change is predicted to cause major shifts in ocean currents confounding migration pathways, habitat suitability and potentially leading to new invasions (Rahel & Olden 2008).
The crown of thorns starfish (Acanthaster planci) is native in the Indo-Pacific region and is not destructive at low population densities. However, irruptive crown of thorns starfish (COTS) population booms are considered a significant threat to coral reefs and are responsible for approximately 42 percent of observed cover loss in the Great Barrier Reef during an irruption (D’eath et al. 2014). Localized nutrient pollution can lead to an increased abundance of COTS and increased sea temperatures is expected to also increase both abundance and range of COTS (Uthicke et al. 2015.) The Australian government is devoting considerable resources (~AUS $150M) into understanding and managing COTS, including significant research on the factors that lead to a population irruption of COTS.
The eradication and control of such species can be particularly challenging in marine environments, and managers often need to rely on lengthy and labor-intensive manual removal (Sutherland et al., 2017). Managers are exploring underwater robotics for controlling certain species. Genetic interventions offer potentially transformative new tools in controlling problematic non-native invasives and irruptive species. Some species and technologies lend themselves to a caged release that keep the engineered individuals from mixing fully into the ecosystem.
BIG IDEA
Initiate the vital proof of concept phase in the development of innovative genetic biocontrol methods for invasive and irruptive marine invertebrates. There are several potential technical research and development pathways for the development of these innovative new approaches to address this particularly difficult conservation and restoration need. While these tools potentially provide a highly targeted and effective means to mitigate the impacts from problem species, the power of these tools will require extensive testing and vetting prior to application in open water marine settings. It should also be noted that these applications are all recommended for caged release timed to have the greatest influence on a potential irruption of species.
The development of genetic interventions raises several particularly profound ethical and regulatory considerations. All of these considerations will require patient and focused engagement with a broad mix of stakeholders. While this Big Idea acknowledges those challenges, it is focused on the development of the techniques for the control of marine invertebrates starting with COTS. If this research were to be successful and gain social acceptance it could have a profound influence on the adoption of genomic interventions.
Specifically, the development of repressible lethal and pheromone disruption systems are promising innovations:
- Repressible lethal systems involve genetic circuits where immature life stages require the presence of a “repressor” molecule (often tetracycline) that prevents a toxic gene from getting expressed. Mature organisms no longer need the repressor and can be released. Offspring resulting from crosses between wild and engineered organisms die in the absence of the repressor20.
- Pheromone disruption systems involve engineering starfish that generate inappropriate signals may disrupt mating behavior and reduce reproductive success. Pheromones are compounds released by organisms that serve as signals to other members of the species. Starfish and other species utilize pheromones to synchronize mating, communicate stress, and form large group aggregates to improve chances of fertilization.
These genetic systems are compatible with caged releases. Transgenic animals will be confined to strategic locations. COTS engineered to continuously release alarm pheromones will act as repellants to protect sensitive areas. COTS engineered to continuously release aggregation pheromones will guide wild COTS to traps for elimination. The repressible lethal system enables lab breeding of COTS, provides a system of transgene biocontainment, and reduces spawning success from mating aggregations.
PROJECT TEAM & PARTNERS
PROJECT TEAM
Crown of Thorns Experts
Russ Babcock, CSIRO, leads the modelling effort of COTS irruptions.
Bernard Degnan, Director of the Centre for Marine Science at the University of Queensland
Scott Cummins, Associate Professor at University of the Sunshine Coast.
John Keesing, University of Western Australia.
David Westcott, CSIRO COTS Specialist.
Synthetic Biologist
Maciej Maselko, CSIRO Synthetic Biology Research Fellow at Macquarie University
PARTNERS
Commonwealth Scientific and Industrial Research Organization, Australia.
Great Barrier Reef Foundation
BUDGET
Phase 1 (Proof of Concept) Budget is $450,000 for 3 Years
RISKS & CHALLENGES
There are limited technical risks in Phase 1 given the number of other species where gene expression and regulatory mechanisms have been identified and manipulated.
Conducting lab work which could one day be used for genome engineering can be controversial
- Mitigation: Engaging the public early on and being transparent about the research and potential intent will be essential.
TIMELINE
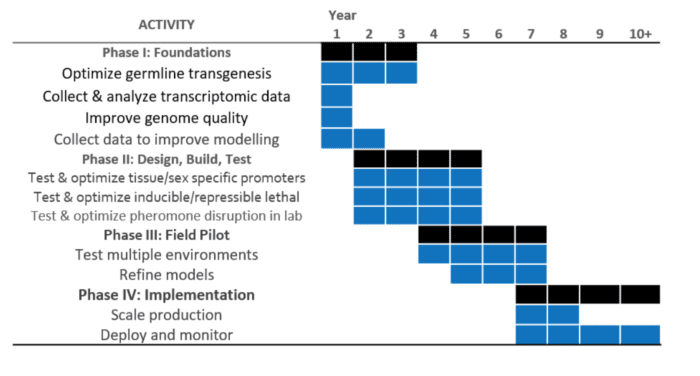
Phase 1: Foundational Research
Under a foundational proof of concept program, initial research will be conducted in order to develop transgenesis and possibly SCNT (cloning) protocols:
- Researchers will establish a rearing facility and capture wild, adult organisms. They would then develop the following:
-
-
- Adapt microinjection techniques from purple sea urchin (widely used as a model organism).
- Test performance of existing tools (e.g.CRISPR-Cas9, Piggybac transposon).
- Test potential for primary tissue culture and somatic cell nuclear transfer for accelerated breeding.
-
- Researchers will collect tissue specific, sex specific, and developmental transcriptomes, which will enable identification of when/where genes are expressed and inform transgene design.
- Collect RNA from multiple tissues and developmental stages, perform next-generation high throughput sequencing and bioinformatic data analysis.
- A combination of short and long read sequencing technologies should be used to improve and refine genomic data for COTS, since existing genomic data is highly fragmented.
- During this foundational stage, life history data on will be collected for modelling biocontrol methods. Accurate modeling of genetic biocontrol requires high quality life-history data on recruitment, spawning frequencies, life-spans, distances traveled, etc. Pheromone dispersal and effective ranges will be determined.
Future Phases
Phase 2: Design, Build, and Lab Test (4 years)
During phase 2, researchers would test and optimize molecular tools including tissue/sex specific promoters and inducible/repressible expression systems:
- Transcriptomic data collected in Phase I would enable identification of where and when genes are expressed. This information would be used to infer the identities of likely regulatory elements enabling engineering.
- Organisms will next be engineered to express reporter genes under the control of candidate promoters for sex/tissue specific expression patterns. Inducible/repressible expression systems used successfully in many other organisms (e.g. tet-on/off) will be tested.
- Optimization of pheromone continuous pheromone production.
- These modified organisms would be tested and refined in a lab setting.
Phase III: Pilot Testing (4 years)
In Phase III, promising transgenic constructs developed in Phase II would be tested under realistic controlled environmental conditions. Candidate locations might include advanced simulated environments (e.g. Biosphere II) and potentially distantly isolated populations of invasive organisms. Transgenic organisms that perform well under idealized laboratory conditions frequently fail under field conditions. Lab and field testing will likely demonstrate that the biocontrol strains will have important differences from the wild type (e.g. sperm counts). These differences will need to be revolved and factored in for genetic biocontrol to be feasible for implementation.
Phase IV: Implementation (4 years- Ongoing)
A Pilot control program should provide a first attempt to at targeted eradication/suppression of pests using genetic biocontrol alone or integrated with existing methods. The best approach is likely to be a multi-year population control project with appropriate untreated control sites. In order to scale, an entity (commercial/non-profit) will need to be formed for mass production of biocontrol organisms and assistance with biocontrol.